Rice nuclear physics team tapped to lead $15 million Large Hadron Collider upgrade project
March 24, 2024
Under the direction of Wei Li, our heavy groups will build time-of-flight detectors for the CMS experiment at CERN. Our groups have vast experience with these types of detector using Multi-Gap Resistive Plain Chambers (MRPCs). What makes this project especially interesting is the use of a new technology, based on Low Gain Avalanche Diodes (LGADs). Accurate timing is all-important for time-of-flight detectors, and LGADs can deliver such timing with a precision of 30 picoseconds. What's more, these detectors are silicon based and thus can be very thin, possibly situated relatively close to the beam line without having too much of an effect on detectors that are mounted further away.
A Rice Media press release can be found at this link.
A Rice Media press release can be found at this link.
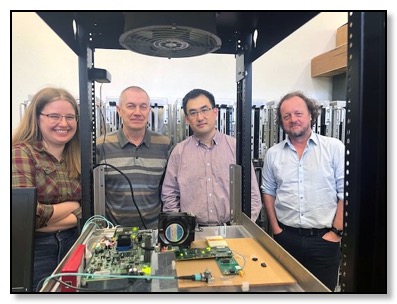
In the spotlight: QuarkNet
September 30, 2023
Our Rice/UH QuarkNet center made it in this week's QuarkNet Friday Flyer. Take a look at this URL: Friday Flyer Sept.28, 2023 or look at the picture, below.
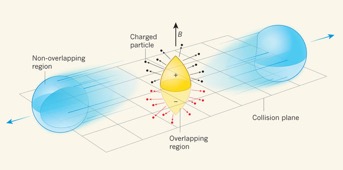
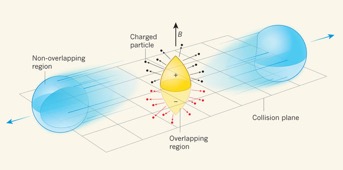
QuarkMatter 2023
September 04, 2023
The QuarkMatter 2023 conference wrapped up with many group pictures. Below is a small collage of pictures, including Yiding Han's presentation.
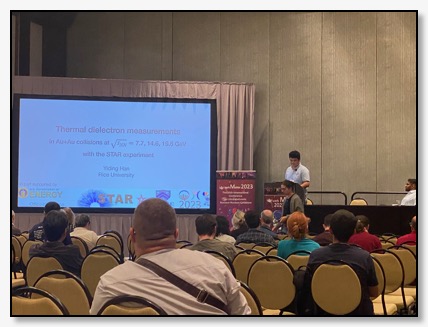
Yiding Han's presentation on BES-2 Thermal Dielectron Measurements
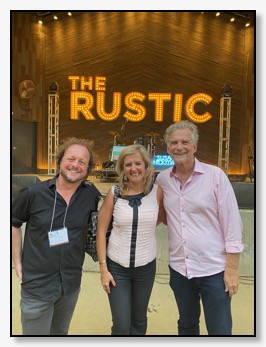
Co-chairs of the QuarkMatter conference at The Rustic
The STAR Collaboration at QM23
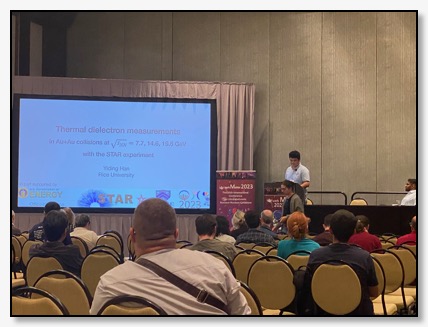
Yiding Han's presentation on BES-2 Thermal Dielectron Measurements
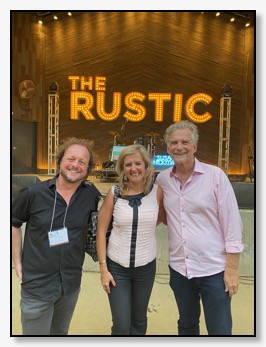
Co-chairs of the QuarkMatter conference at The Rustic
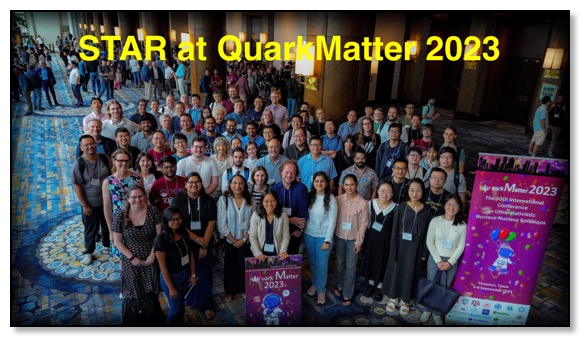
The STAR Collaboration at QM23
STAR PreQM'23 Meeting
September 02, 2023
Almost 70 STAR collaborators descended on the Rice campus to participate in the Pre-QuarkMatter meeting. Within a 3-day, jam-packed agenda the collaboration rehearsed all its oral contributions to the QuarkMatter conference, which followed the next week. While outdoor temperatures were consistently about 100F, the campus provided for a welcoming environment in part thanks to the graduate student bar Valhalla and the neighboring Rice Village. Of course, no self-respecting workshop can end without a group picture and below, you'll see an energized STAR collaboration in the Kyle Morrow room on the 3rd floor of the Fondren Library at Rice University. On the big screen you will recognize a few of our online participants, and all the way in the front you can see some of our (very) junior, future collaborators. 😀
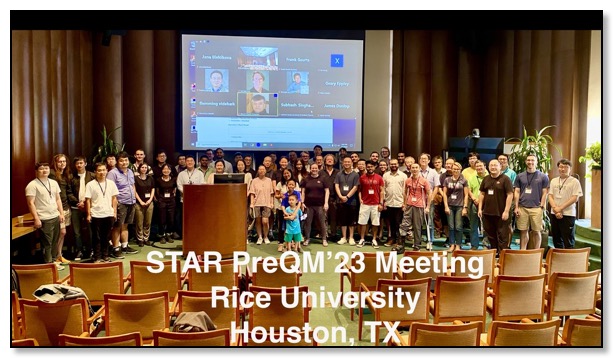
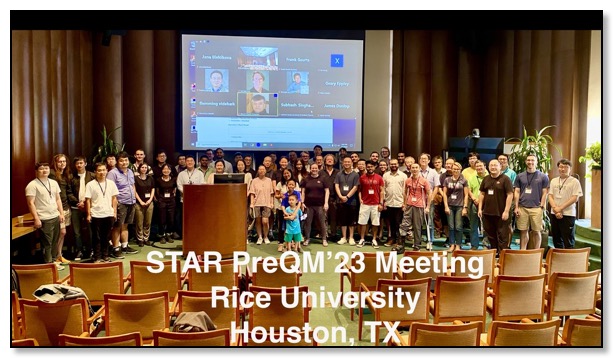
Rice Thresher interview
August 23, 2023
The Rice Thresher is consistently listed in Princeton Review's top-10 of best college newspapers. I was honored to get an online feature. Many thanks to Molly Kyles for a great conversation and her professional work!
https://www.ricethresher.org/article/2023/08/frank-geurts-to-lead-worlds-longest-running-nuclear-collider-experiment
Frank Geurts to lead world’s longest running nuclear collider experiment
By Molly Kyles 8/22/23 11:41pm
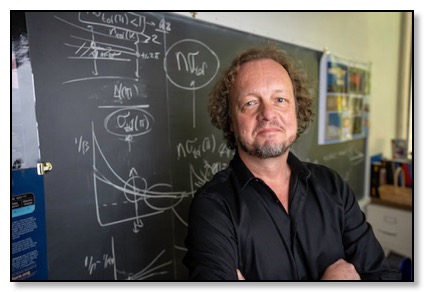
Rice University physicist Frank Geurts has been named co-spokesperson for the Solenoidal Tracker at Relativistic Heavy Ion Collider collaboration, the world’s first heavy-ion collider located at Brookhaven National Laboratory. Alongside Brookhaven Lab physicist Lijuan Ruan, Geurts will lead STAR for the next three years to collect data on nuclear collisions and study the matter that was present at the origin of the universe.
“The neutrons and protons inside atoms melt into their substructure: quarks and gluons,” Geurts said. “We slam nuclei to smithereens and look at what comes out of it. [We] deduce what we had in [early universe] conditions.”
The STAR experiment can replicate the extreme conditions that existed just microseconds after the Big Bang with detailed control over the data collected.
“In the lab, you can have these kinds of collisions many, many times. You can study the conditions and properties created in a lab in a very detailed way,” Ruan said.
For Geurts, these questions surfaced in his life earlier than most. The Netherlands-born scientist can trace his love for physics back to the stars.
“As a kid, I was always very interested in astronomy. I woke up at two o’clock in the morning, looking at the stars,” Geurts said. “The rabbit hole was there, I just went deeper and deeper, and figured that nuclear particle physics was my thing. There was this drive in asking, I went from the large objects in the sky to zooming in and understanding why things are what they are.”
Geurts went on to study physics in his undergraduate and graduate years in the Netherlands. During that time, Geurts had the opportunity to work at CERN, the European Organization for Nuclear Research, before applying for a postdoctoral position at Rice and beginning his involvement with STAR.
“I [selected] Rice because [of] its reputation in designing, development and research in detectors for particle physics,” Geurts said. “I grew into [the STAR] experiment. I was a co-convener, then I was a Physics Analysis Coordinator, then I was a deputy spokesperson and as of recently, I am, together with Lijuan, a co-spokesperson.”
Ramamoorthy Ramesh, Rice’s vice president of research, emphasized the significance of Rice’s affiliation with STAR.
“At the end of the day, you want our faculty to be competing for the biggest discoveries. And the biggest discoveries invariably mean the biggest recognition,” Ramesh said. “We need to be a part of that big conversation, where discoveries are being made.”
Ramesh analogizes the scope of scientific discovery to the vastness of a boba tea shop menu.
“The origins are still being discovered. In the beginning there were just protons, electrons, neutrons,” Ramesh said. “Now, we know there are maybe 33 subatomic particles, with different flavors. It’s exactly like going to a boba tea shop. It’s just tea with boba, but there are 60 different varieties.”
The public funding that the STAR collaboration relies on requires its findings to be communicated to the general public.
“We’re the longest running nuclear colliding experiment. That is, to a substantial degree, thanks to taxpayers. We need to make sure that high school kids, undergrads and middle school [students] know the science so they can have the same enthusiasm we have,” Geurts said.
STAR plans to record its final collisions in 2025, before being converted into an electron-ion collider. However, STAR’s work will continue after its last collision.
“STAR will likely continue another five or eight years just digesting and analyzing,” Geurts said. “We basically redesign a new experiment each time we look at the data.”
From a curiosity in the stars to colliding particles at super speeds, for Geurts, all discoveries come back to collaboration.
“To students that want to make big discoveries, talk about science,” Geurts said. “Discuss it with your peers, with your professors, discuss it with your parents for all I care. Big discoveries come not out of single minds. Big discoveries come out of humans talking to each other.”
https://www.ricethresher.org/article/2023/08/frank-geurts-to-lead-worlds-longest-running-nuclear-collider-experiment
Frank Geurts to lead world’s longest running nuclear collider experiment
By Molly Kyles 8/22/23 11:41pm
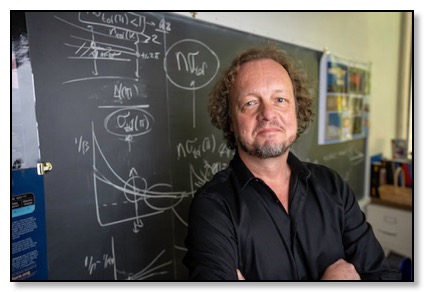
Rice University physicist Frank Geurts has been named co-spokesperson for the Solenoidal Tracker at Relativistic Heavy Ion Collider collaboration, the world’s first heavy-ion collider located at Brookhaven National Laboratory. Alongside Brookhaven Lab physicist Lijuan Ruan, Geurts will lead STAR for the next three years to collect data on nuclear collisions and study the matter that was present at the origin of the universe.
“The neutrons and protons inside atoms melt into their substructure: quarks and gluons,” Geurts said. “We slam nuclei to smithereens and look at what comes out of it. [We] deduce what we had in [early universe] conditions.”
The STAR experiment can replicate the extreme conditions that existed just microseconds after the Big Bang with detailed control over the data collected.
“In the lab, you can have these kinds of collisions many, many times. You can study the conditions and properties created in a lab in a very detailed way,” Ruan said.
For Geurts, these questions surfaced in his life earlier than most. The Netherlands-born scientist can trace his love for physics back to the stars.
“As a kid, I was always very interested in astronomy. I woke up at two o’clock in the morning, looking at the stars,” Geurts said. “The rabbit hole was there, I just went deeper and deeper, and figured that nuclear particle physics was my thing. There was this drive in asking, I went from the large objects in the sky to zooming in and understanding why things are what they are.”
Geurts went on to study physics in his undergraduate and graduate years in the Netherlands. During that time, Geurts had the opportunity to work at CERN, the European Organization for Nuclear Research, before applying for a postdoctoral position at Rice and beginning his involvement with STAR.
“I [selected] Rice because [of] its reputation in designing, development and research in detectors for particle physics,” Geurts said. “I grew into [the STAR] experiment. I was a co-convener, then I was a Physics Analysis Coordinator, then I was a deputy spokesperson and as of recently, I am, together with Lijuan, a co-spokesperson.”
Ramamoorthy Ramesh, Rice’s vice president of research, emphasized the significance of Rice’s affiliation with STAR.
“At the end of the day, you want our faculty to be competing for the biggest discoveries. And the biggest discoveries invariably mean the biggest recognition,” Ramesh said. “We need to be a part of that big conversation, where discoveries are being made.”
Ramesh analogizes the scope of scientific discovery to the vastness of a boba tea shop menu.
“The origins are still being discovered. In the beginning there were just protons, electrons, neutrons,” Ramesh said. “Now, we know there are maybe 33 subatomic particles, with different flavors. It’s exactly like going to a boba tea shop. It’s just tea with boba, but there are 60 different varieties.”
The public funding that the STAR collaboration relies on requires its findings to be communicated to the general public.
“We’re the longest running nuclear colliding experiment. That is, to a substantial degree, thanks to taxpayers. We need to make sure that high school kids, undergrads and middle school [students] know the science so they can have the same enthusiasm we have,” Geurts said.
STAR plans to record its final collisions in 2025, before being converted into an electron-ion collider. However, STAR’s work will continue after its last collision.
“STAR will likely continue another five or eight years just digesting and analyzing,” Geurts said. “We basically redesign a new experiment each time we look at the data.”
From a curiosity in the stars to colliding particles at super speeds, for Geurts, all discoveries come back to collaboration.
“To students that want to make big discoveries, talk about science,” Geurts said. “Discuss it with your peers, with your professors, discuss it with your parents for all I care. Big discoveries come not out of single minds. Big discoveries come out of humans talking to each other.”